All published articles of this journal are available on ScienceDirect.
The Effectiveness of N-acetylcysteine in Alleviating Kidney Dysfunction in Ifosfamide-treated Rats
Abstract
Background:
Renal damage and dysfunction are possible complications of pharmacotherapy with ifosfamide (IF), which also manifests urotoxic properties. A routine drug used to reduce the risk of IF-induced cystitis is mesna. Compounds with effect expected to be similar to mesna include N-acetylcysteine (NAC).
Objective:
The objective of the paper was histopathological verification of the uroprotective effect of NAC and assessment of whether this effect is accompanied by a potential nephroprotective effect.
Methods:
The experiment was conducted on 3 groups: 1 – control, sham-treated rats, 2 – animals treated with 5 times the IF dose administered i.p. (50 mg/kg b.w.) and 3 – rats treated with 5 times the IF dose administered i.p. + NAC administered p.o. (200 mg/kg b.w.). The renal function was evaluated analysing classical and new protein parameters (cystatin C - CysC, kidney injury molecule-1 – KIM-1 and nephrin - NPH) in blood and urine. Furthermore, histopathological analysis of bladders and kidneys was carried out.
Results:
Treatment with IF resulted in the development of cystitis, with no significant histopathological disturbances in the kidneys, and caused an increase in concentration and 24-hour excretion of CysC, KIM-1 NPH in the urine. Additional NAC administration caused a reduction of the said biochemical disturbances as well as improvement of the histopathological image of the urinary bladders.
Conclusion:
The IF therapy caused cystitis and kidney dysfunction of functional tubulopathy and early glomerulopathy character. Additional administration of NAC entailed improvement in the urinary bladder morphology and renal function. NAC is, thus, a compound exerting both uro- and nephroprotective effects.
1. INTRODUCTION
Damage of the kidneys, as an essential organ eliminating xenobiotics, is a possible adverse effect of multiple medicinal substances. Many drug classes are characterised with potential nephrotoxicity. These include anti-infectives (aminoglycoside antibiotics, amphotericin B, sulphonamides), anti-retrovirus agents, non-opioid analgesics, immunosuppressants, contrast agents, agents containing heavy metals, statins, proton-pump inhibitors, anticonvulsants, biphosphonians, angiotensin-converting-enzyme inhibitors, AT1-receptor antagonists for angiotensin II, loop and thiazide diuretics, antiplatelet thienopyridines and other [1, 2]. Herbal preparations can also impair renal functions (“Chinese herbs” containing aristolochic acid) [3, 4]. Furthermore, a significant class of drugs demonstrating nephrotoxicity are antineoplastic chemothera- peutics, including cyclophosphamide (CP) and ifosfamide (IF) [5, 6].
Those compounds are prodrugs and undergo metabolic biotransformation by CYP3A4, CYP2B6 and CYP2C9 isoenzymes leading to the subsequent production of the active nitrogen mustard, which exerts cytotoxic activity by DNA crosslinking. The process of hepatic and extrahepatic biotransformation of both CP and IF also causes the formation of other by-products, determining toxicities of those drugs, such as acrolein and chloroacetaldehyde (CAA) [7, 8]. Acrolein (2-propenal) is a highly reactive unsaturated aldehyde disturbing the cell redox balance leading to increased generation of reactive oxygen species synthesis and glutathione depletion. Moreover, it reacts with cysteine, lysine and histidine residues of many proteins, causing the addition of the aldehydic carbonyl group to their structure. The cellular carbonylated proteins are involved in many cellular dysfunctions, including energy production deficiency and loss of cytoskeletal integrity [9]. CAA, like acrolein, is also a factor initiating severe oxidative stress and reacts with protein and non-protein sulfhydryl groups of many compounds, thereby impairs proliferation and apoptosis regulatory mechanisms. CAA also inhibits lysosomal cysteine proteases (e.g., cathepsin B), resulting in disturbed digestion of endocytosed proteins. Moreover, CAA-induced depletion of reduced glutathione diminishes the capacity of the cells to scavenge free radicals, thereby increasing their susceptibility to oxidative stress [10].
Acrolein, excreted with urine, is a compound considered to damage mainly the urinary bladder, whereas CAA impairs kidneys, mostly affecting proximal tubules. Due to the fact that both CP and IF share the same metabolic pathways during their biotransformation processes, both compounds are considered to be uro- and nephrotoxic drugs [11, 12]. However, there are some quantitative differences in the metabolism of CP and IF – during IF biotransformation, the higher formation of CAA takes place, hence this drug is seen as more nephrotoxic compared to CP [8]. To reduce the risk of these adverse effects, during CP or IF therapies, forced diuresis is applied and compounds with uro- and nephroprotective effects are administered, such as mesna (sodium 2-mercaptoethane- sulfonate). It is a compound reducing the excessive oxidative stress and the resulting cellular oxidative damage caused by acrolein and CAA and rebuilding the glutathione resources in the cells [13]. A compound characterised, similarly to mesna, with the presence of sulfhydryl groups, essential from the point of view of glutathione regeneration and antioxidating effective, is N-acetylcysteine (NAC), currently applied as a secretolytic agent in obstructive conditions of the respiratory tract and as an antitoxin in paracetamol poisoning [14]. The registered indications for administration of this drug do not, however, include its application as a protective agent in IF therapies [15].
Thus, the objective of our experiment was the assessment of the nature of kidney dysfunction in rats treated with IF as well as verification of the expected alleviating, uro- and potential nephroprotective effect of NAC. Our assessment was based on the microscopic evaluation of kidneys and urinary bladders as well as laboratory analysis of classical parameters evaluating the renal functions and new protein markers of kidney functions: cystatin C (CysC), kidney injury molecule-1 (KIM-1) and nephrin (NPH). These compounds are modern indicators of kidney injury, and their increased occurrence in urine precedes obvious functional and structural kidney dysfunction.
2. MATERIALS AND METHODS
2.1. Animals Used in the Experiment
In the experiment, 10-week Wistar laboratory rats (n=36) of average initial body weight (b.w.) of 305.38 ± 84.56 [g] were used, with the same number of males (n=18; with average initial b.w. 388.67 ± 28.06 [g]) and females (n=18; with average initial b.w. 221.58 ± 10.83 [g]), obtained from the central breeding animal lab of the Faculty of Pharmacy of the Jagiellonian University Medical College in Krakow. The experiment was carried out according to the “3R” rules effective in biomedical sciences, including but not limited to ensuring the welfare of the examined animals [16].
2.2. Housing and Husbandry
Upon transport to the animal lab of the Experimental and Innovative Medicine Centre, the studied rats remained in collective one-sex cages, 2-3 individuals per cage, with dust-free litter and wooden environmental “enrichments” (according to the “3R” rules) as well as free access to feed (LABOFEED B, Kcynia, Poland) and water. In the living space, constant conditions were maintained and monitored: temperature (20-24 oC), air humidity (60-70%), air ventilation (providing full air exchange in 8-10 hours), lighting (130-325 lux with 12/12 hour day/night cycle) and noise level < 30 dB. After 10 days of quarantine, the rats were randomly assigned to the studied groups, with an equal number of individuals in each group (n=12) and maintaining their gender structure. The experiment was conducted in the light phase of the day and the procedures described below were performed in the same morning hours, ending before noon.
2.3. Study Groups
3 groups (each n=12) of examined rats were studied: group 1 – control - consisted of healthy individuals, not treated with any pharmacological agents, subjected only to a sham therapy with some saline solution administered i.p.. Group 2 comprised animals treated with 5 consecutive doses of IF administered i.p.. In group 3, in addition to i.p. administration of IF, NAC was applied p.o.
2.4. Ifosfamide Administration
For consecutive 5 days, animals from group 2 and 3 received IF (Holoxan® 1g; Baxter Oncology GmbH; phials containing powder for preparation of a solution for injections) in the dose of 50 mg/kg b.w./day administered intraperitoneally. The IF dose used in the experiment complies with the literature data and description of other experiments on rats using IF [17, 18].
2.5. N-acetylcysteine Administration
One hour after the administration of each IF dose, animals from group 3 were administered NAC per os in the dose of 200 mg/kg b.w./day (Acetylcysteine Sandoz® 100mg/ml; ampules a' 3 ml containing 300 mg of NAC). The proper volume of the NAC solution containing the calculated dose was added to ca. 1 ml of preparation Nutridrink® (Nutricia, Poland) and administered in rats using a tuberculin syringe. Due to its sweet taste, animals eagerly ate NAC administered this way. The NAC dose used in the experiment is consistent with reports from other experimental studies with laboratory rats and the applied route of administration of that compound [19, 20]. It is believed that NAC administered within the range of 100-500 mg/kg is the dose that is among the most frequently applied in experimental studies, causing no toxic effects [21].
2.6. Monitoring in Metabolic Cages
Upon administration of the last doses of physiological saline or drugs, the tested animals were placed in individual metabolic cages with unlimited access to feed and water in order to measure the daily diuresis as well as water and feed intake for 24 hours. After that period, the final body weight was taken, the above parameters were determined, and the pH of urine was checked with dipstick tests (Insight, ACON Laboratories). The obtained urine samples were centrifuged and frozen at -80 oC until analytical determinations are made.
2.7. Blood Collection, Organ Procurement and Animal Euthanasia
Having completed the monitoring in the metabolic cages, the rats were put under deep general anaesthesia with preliminary inhalation anaesthesia (isoflurane) deepened with intraperitoneal administration of pentobarbital (Morbital®, Biowet Puławy, Poland) in the doses of 50 mg/kg b.w. In this state, thoracotomy was performed and blood was sampled from the heart. The collected blood samples were centrifuged at 3000 rpm for 10 minutes and the obtained serum was frozen in -80oC for biochemical testing to be performed in the future. After blood was collected, the rats received an additional, lethal dose of pentobarbital: 100 mg/kg b.w. Finally, laparotomy with bilateral nephrectomy and cystectomy was performed. The harvested organs were stored in containers with 4% formaldehyde solution in PBS.
2.8. Parameters Determined in Serum
In the obtained serum samples, using the automatic analyser Siemens Advia 1200, concentrations of classical parameters reflecting renal function were determined: creatinine [mg/dL], urea [mg/dL] (along with conversion to BUN [mg/dL] and the BUN/creatinine quotient), uric acid [mg/dL], total protein [g/dL] and electrolytes (sodium, potassium and chlorides; [mmol/L]. Moreover, in serum samples, the concentration of CysC [µg/mL] was determined using the immunoenzymatic assay (ELISA) by means of the ELISA kit by SunRedBio (Shanghai Sunred Biological Technology Co., Ltd, China; catalogue number DZE201110 146) and strictly following the manufacturer's instructions.
2.9. Parameters Determined in Urine
With the ELISA method, in the obtained urine samples, the concentrations of 3 protein markers of renal function were determined: CysC (µg/mL), kidney injury molecule (KIM-1) (pg/mL) and NPH (ng/mL), using ELISA kits (catalogue numbers DZE201110146, DZE201110550 and DZE201111424, respectively) made by SunRedBio (Shanghai Sunred Biological Technology Co., Ltd, China) and strictly following the manufacturer's instructions. Moreover, the concentrations of the aforementioned compounds were converted on the basis of determined diuresis to their 24-hour excretion with urine, expressing them in (µg/24h), (pg/24h) and (ng/24h), respectively.
2.10. Histopathological Evaluation
The histopathological assessment was performed on tissues harvested from rats, and included kidney and bladder. Tissues sections fixed in 10% buffered formalin were dehydrated by means of graded ethanol and xylene baths and embedded in paraffin wax. Sections of 3-4 µm were stained with haematoxylin and eosin (HE). The detailed methodology for making preparations has been described in one of our papers published recently [22]. General histopathological examination was evaluated at magnification of 10x, 40x and 100x (objective lens) and 10x (eyepiece) and photographic documentation at magnification of 10x (objective lens) and 10x (eyepiece) was made. The microscopic evaluation was performed in a blinded fashion, using a standard light microscope Olympus BX41 and CellSens software (Olympus Corporation, Tokyo, Japan).
2.11. Statistical Analysis
The obtained results were analysed with statistical software package Statistica ver. 12 Statsoft. The basic statistics of location and variability were calculated preliminarily (mean, standard deviation, median, the scope of the first and third quartile). Subsequently, the normal distribution of the obtained results was investigated with the Lilliefors test and the Shapiro-Wilk test. It was assumed that the results of both tests must be consistent in terms of normality tests. When the results were divergent, the distribution was assumed to be abnormal. The next step of statistical analysis consisted of preliminary assessing differences in a given parameter in all analysed groups (1-2-3). If the analysed parameters have a normal distribution, the one way ANOVA test was applied, followed by post-hoc comparison of groups 1-2 and 2-3 with Student's t-test. Parameters demonstrating lack of normal distribution were analysed with Kruskal–Wallis test followed by post-hoc comparison using Mann–Whitney test. Statistical significance was assumed at P≤0.05. We assessed differences in paired groups 1-2 to determine the initial changes induced by IF treatment and 2-3 to show changes in animals additionally treated with NAC. Groups 1 and 3 were not compared, as the purpose of our experiment was to assess the effect of NAC on kidney and bladder function and morphology in IF treated animals, not in healthy individuals.
The results were provided using arithmetic mean and standard deviation.
3. RESULTS
3.1. Animals Lost in the Study and Adverse Effects Observed During the Experiment
All rats from group 1 completed the experiment. In group 2, two individuals died with symptoms of cachexia before they were administered the last IF dose, most probably due to the adverse, systemic effect of the administered drug. In the group additionally treated with NAC, there was 1 such individual. Therefore, we eventually analysed the obtained results in groups with the following number of individuals: group 1: n=12, group 2: n=10, and group 3: n=11. In group 2 and 3 rats, we observed a bristled coat in most individuals compared to group 1.
3.2. 24-hour Monitoring in Metabolic Cages – Vital Parameters
All vital parameters assessed in individual animal groups were not significantly different, except for daily feed intake, which was significantly lower in group 2 animals compared to group 1. Towards the end of the experiment, the rats additionally treated with NAC to a slight degree tended to have heavier body weight and higher daily feed intake compared to the rats not treated with NAC. Detailed results are presented in Table 1.
3.3. Parameters Determined in Serum
For most classic parameters reflecting renal function, no significant intergroup differences were found except for urea, BUN and total protein concentration, which were significantly higher in group 2, compared to group 1. In addition, blood CysC was also significantly higher in individuals from group 2 comparing to those ones from group 1. In group 3, concentrations of urea and BUN were significantly lower compared to group 2. Detailed results are presented in Table 2.
3.4. Parameters Determined in Urine
Analysis of parameters determined in urine revealed significant intergroup differences. Urine pH did not differ in the analysed groups, but group 3 showed a tendency towards urine alkalinisation compared to both other groups. The concentrations of CysC, KIM-1 and NPH determined in urine were ca. 4 times higher in group 2 than in controls from group 1. On the other hand, NAC treatment in group 3 led to a significant decrease in concentrations of all the above-mentioned proteins, comparing to group 2. Detailed values are presented in Table 3.
Analogously to concentrations in urine, daily excretion of CysC, KIM-1 and NPH in urine was significantly, ca. 4 times, higher in group 2 individuals compared to the healthy rats from group 1. NAC treatment implemented in group 3 reduced CysC, KIM-1 and NPH excretion in urine to values being ca. 48%, 44% and 37%, respectively, of those found in group 2. Detailed values are presented in Fig. (1).
Group 1 | Group 2 | Group 3 | |
---|---|---|---|
Initial body weight [g] |
312.63 ± 97.67 | 301.38 ± 84.56 | 305.10 ± 92.36 |
Final body weight [g] |
330.38 ± 87.34 | 274.25 ± 100.73 | 290.75 ± 109.75 |
24-hour water intake [mL/24h] |
11.67 ± 5.72 | 8.75 ± 6.27 | 9.00 ± 5.66 |
24-hour feed intake [g/24h] |
23.67± 5.01 | 12.00 ± 7.98a& | 18.00 ± 7.40 |
24-hour diuresis volume [mL/24h] |
3.80 ± 2.49 | 3.38 ± 1.92 | 3.60 ± 1.82 |
Group 1 | Group 2 | Group 3 | |
---|---|---|---|
Creatinine [mg/dL] |
0.24 ± 0.04 | 0.25 ± 0.04 | 0.21 ± 0.44 |
BUN [mg/dL] |
18.50 ± 3.34 | 21.86 ± 2.10a* | 18.38 ± 3.66b* |
Urea [mg/dL] |
37.15 ± 4.86 | 46.84 ± 4.50a# | 39.38 ± 7.85b* |
BUN/urea ratio |
78.00 ± 17.83 | 88.67 ± 24.48 | 87.71 ± 14.66 |
Uric acid [mg/dL] |
1.63 ± 0.68 | 1.06 ± 0.59 | 1.15 ± 0.69 |
Total protein [g/dL] |
4.73 ± 0.35 | 5.70 ± 0.19a# | 5.30 ± 0.19 |
Na [mmol/L] |
147.63 ± 0.92 | 148.25 ± 3.11 | 146.00 ± 2.00 |
K [mmol/L] |
5.82 ± 0.70 | 6.38 ± 1.18 | 5.50 ± 1.00 |
Cl [mmol/L] |
104.88 ± 0.84 | 106.00 ± 2.27 | 104.00 ± 2.56 |
CysC [µg/mL] |
0.84 ± 0.29 | 3.48 ± 0.41a# | 2.86 ± 0.79 |
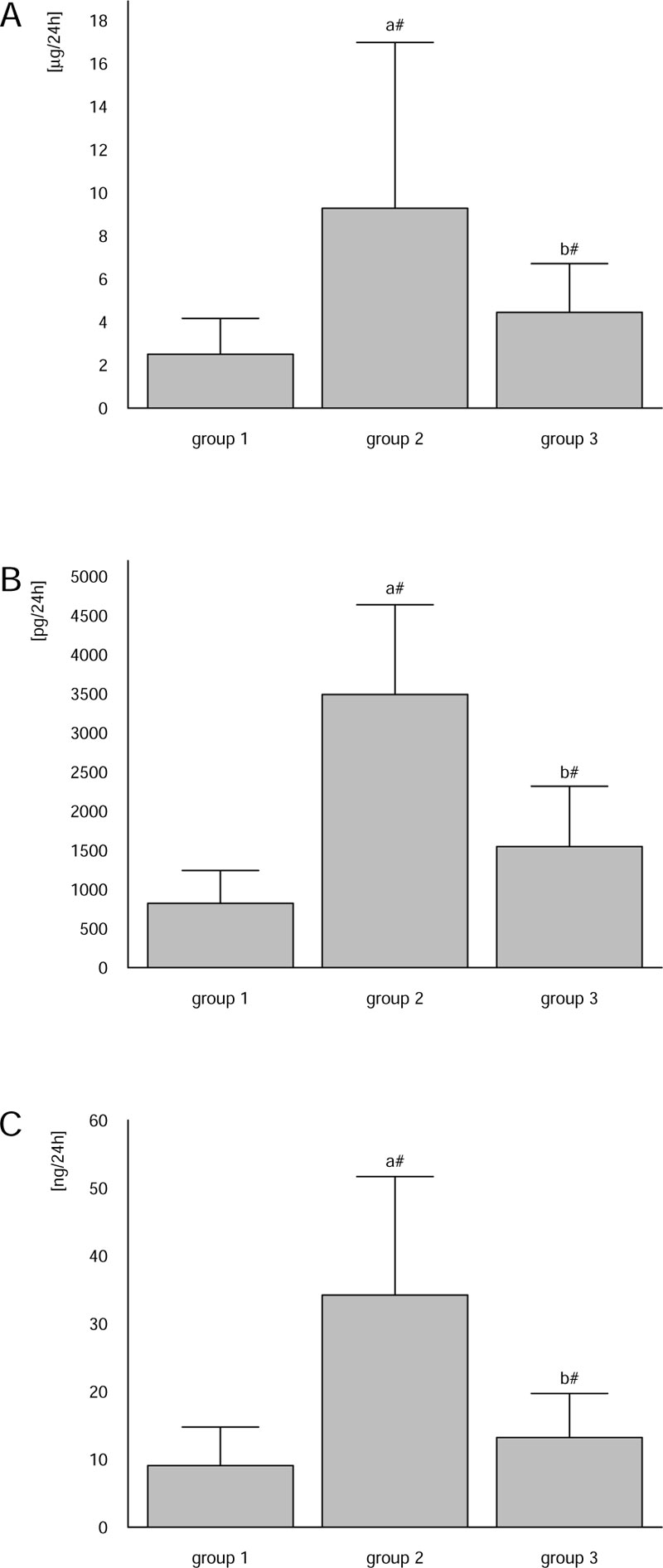
Group 1 | Group 2 | Group 3 | |
---|---|---|---|
pH | 6.80 ± 0.44 | 6.92 ± 1.28 | 7.80 ± 0.27 |
CysC [µg/mL] |
0.63 ± 0.12 | 2.39 ± 1.00a# | 1.32 ± 0.25b# |
KIM-1 [pg/mL] |
211.73 ± 41.29 | 864.34 ± 369.24a# | 466.22 ± 72.28b# |
NPH [ng/mL] |
2.34 ± 0.51 | 8.83 ± 3.27a# | 4.02 ± 0.77b# |
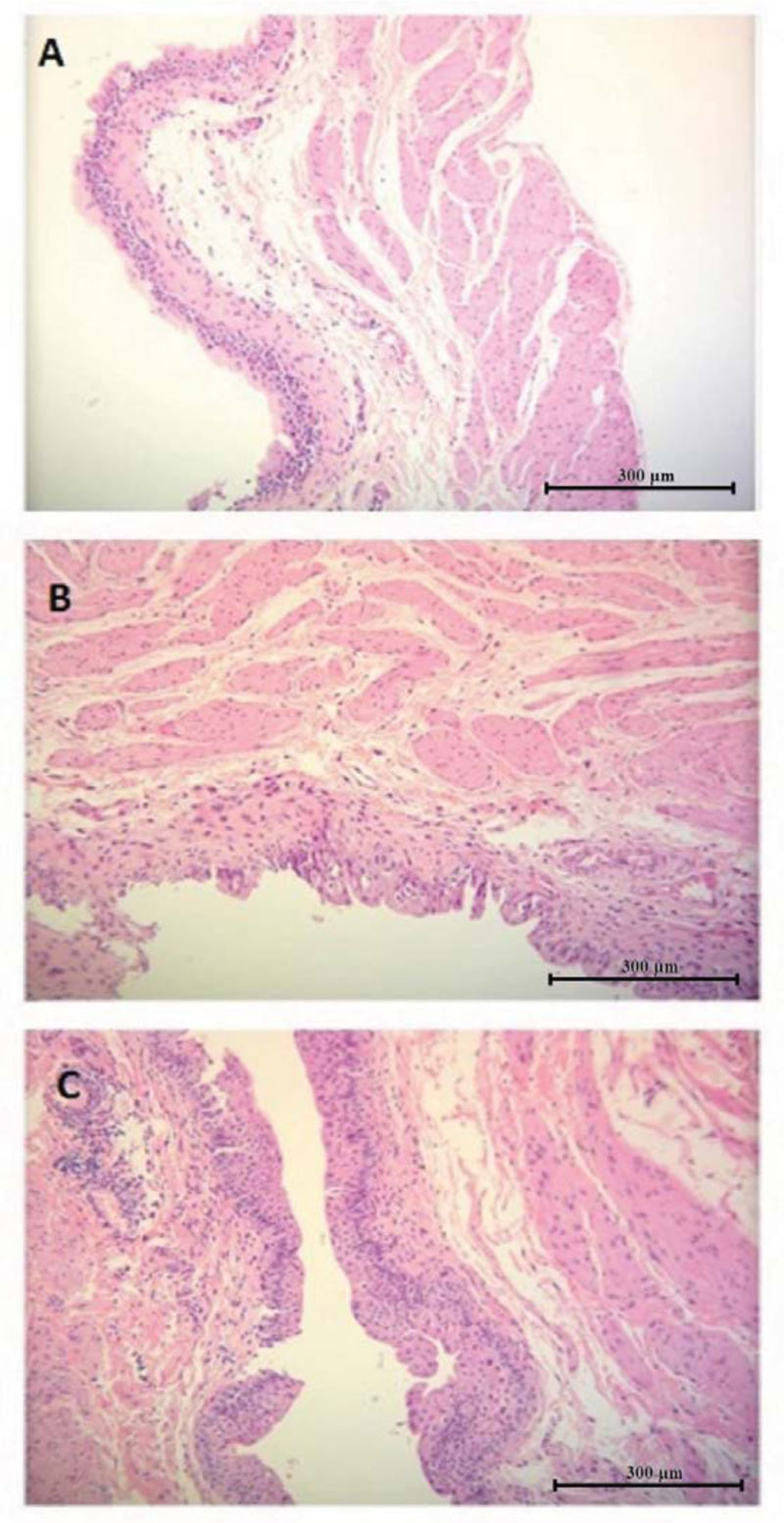
3.5. The Histopathological Assessment of Urinary Bladders and Kidneys
The histopathological image of urinary bladders of rats from group 2, treated exclusively with IF, in comparison to the specimens collected from healthy animals from group 1, revealed slight oedema of the mucosa and submucosa, with minor hyperaemia of the mucous membrane vessels accompanied with moderate hyperplastic and hypertrophic features of the epithelial cells. The cells were partially in columnar arrangement; their polymorphism and anisocytosis were visible. A slight increase in cellularity in the mucous stroma indicates the infiltration of mononuclear cells, mostly with lymphocytic morphology. Moderate oedema of the connective tissue of the muscular membrane of the urinary bladder was also visible. The microscopic images of urinary bladders collected from animals from group 3, additionally treated with NAC, showed an improvement in morphology, compared to those ones from group 2. No significant features of mucosa and submucosa oedema were found. The mucosa epithelium consisted of 5-6 layers, with minor features of hyperplasia, however without features of hypertrophy or cell dysplasia, with accompanying minor anisocytosis and excitation of nuclei, indicating features of renewal. Although smaller in comparison to group 2, the infiltration of mononuclear cells, mostly with lymphocytic morphology, was still present in the mucous stroma.
The microscopic image of urinary bladders in the investigated groups is presented in Fig. (2).
In the kidney preparations from group 2 animals, exfoliation of the apical parts of the cytoplasm of renal tubule epithelia, with their accumulation in the tubular lumen, was observed. However, no features of degeneration or necrosis were revealed. No other significant changes indicating specific pathological changes in the corpuscle, tubules, stroma or vessels of the discussed organ were found.
The image of kidneys of the rats from group 3 was nearer the norm. Slight oedema of the epithelial cells was prevalent over their exfoliation, especially in the apical part of the tubules from the lumen side. This proves the lower impact of IF in the presence of NAC on the tubular cells in comparison to group 2. Moreover, the nuclei of epithelial cells of the tubules were excited, they were euchromatic with chromatin margination and small single nuclei. The tubular lumen was normal, with no congested mass. No hyperaemia was found in the renal parenchyma or glomerular vessels. In both examined groups, morphotic changes in the kidneys probably had no effect on their functionality. The microscopic image of kidneys in the investigated groups is presented in Fig. (3).
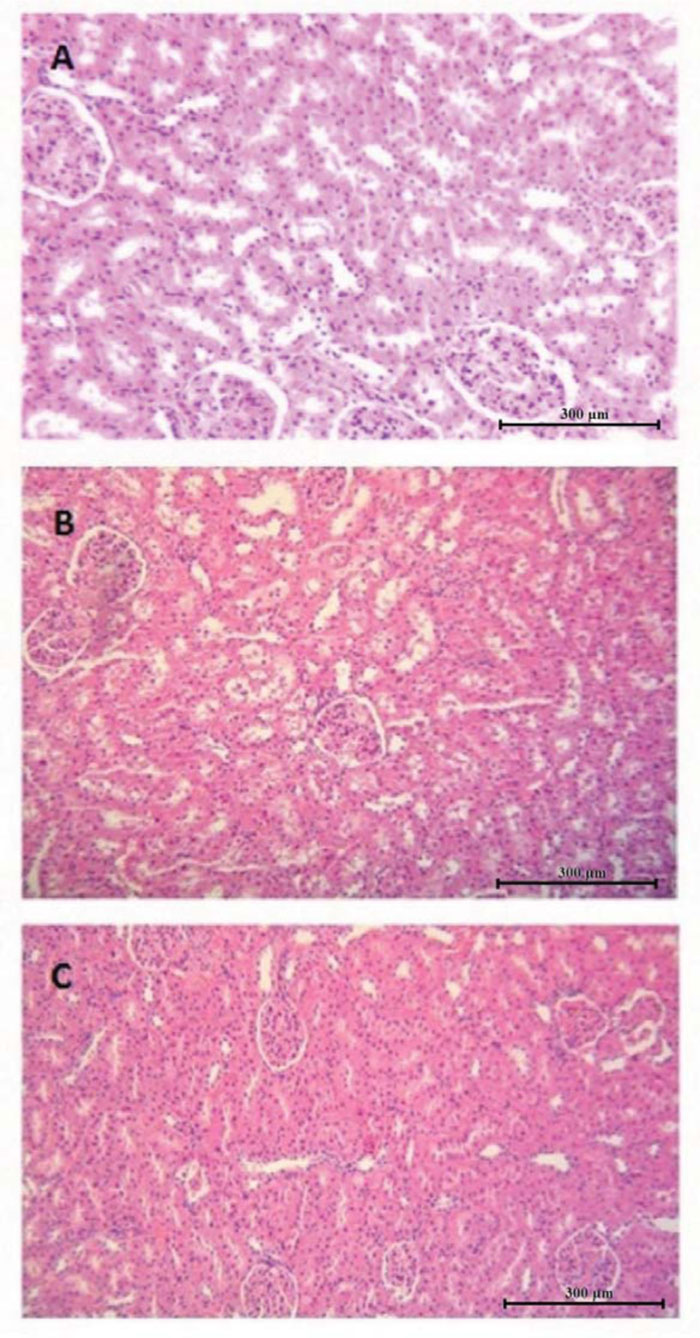
4. DISCUSSION
The analysis of the results obtained in our experiment shows that the administration of IF to rats at the total dose of 250 mg/kg b.w., divided into 5 daily doses, resulted in the development of urinary bladder inflammation, without significant effect on the structure of the kidneys visible in histopathological analysis, but with functional disorders of those organs. Both the 24-hour diuresis value and urine pH did not differ significantly in the analysed groups, even though literature data mention the possibility of renal tubular acidosis and nephrogenic diabetes insipidus during IF therapies. At the same time, literature reports indicate that IF is a potential etiological factor of damage to proximal tubules and development of tubulopathy, which clinically manifests with a wide spectrum of abnormalities and may lead to Fanconi syndrome [5, 23]. The pathogenesis of IF-induced tubulopathy, as mentioned in the Introduction, is related to the emerging IF metabolite – chloroacetaldehyde, mostly synthesised with hepatic CYP3A4, CYP2B6 and CYP2C9 isoenzymes [7, 8], but also by renal isoenzymes CYP3A4, CYP3A5 and CYP2B6 [24]. As mentioned in the Introduction, CAA is a compound disturbing the mitochondrial function, causing energy deficiency, increased tubules cell sensitivity to the oxidative stress and damaging their structure [10, 25]. Furthermore, CAA impairs intracellular calcium homeostasis in proximal tubules due to the inhibition of the Na+/Ca2+ exchanger and affecting the cyclic adenosine monophosphate and protein kinase A signalling. It results in the progressive tubular cell dysfunction and their final necrosis [26].
The results obtained by us regarding CysC, KIM-1 and NPH confirm the hypothesis of the development of functional tubulopathy, without any pathomorphological changes visible at the level of classical light microscopy. The results of classical parameters, determined routinely to estimate the kidney functions, were ambiguous. The creatinine and electrolyte concentration was comparable in all groups. Elevated urea and BUN concentration was demonstrated in group 2 in relation to the control 1 group. Taking into account the serum creatinine level within the norm in all study groups, this growth could reflect increased protein catabolism. However, in individuals from group 2, the parallel increase in total protein concentration in blood was demonstrated. This finding, therefore, negated the hypothesis of protein hypercatabolism. The tendency of increase in the value of the BUN/creatinine quotient in groups 2 and 3 in comparison with group 1 was noticeable (nevertheless, with no statistical significance). This parameter is an azotemia discriminator and its elevated value suggests a hypercatabolic state or prerenal disturbances, such as hypovolemia and decreased renal perfusion [27]. Reduced kidney perfusion could explain the increase of CysC in serum in rats treated with IF. Nevertheless, the value of daily diuresis in all groups was comparable - no oliguria was found in group 2 or 3. Furthermore, as mentioned above, the creatinine level in all groups did not differ significantly, which excludes disturbances in glomerular filtration. We also did not perform any imaging of the kidneys that would allow to verify renal perfusion. Therefore, the results obtained in the serum do not allow for a clear interpretation.
The hypothesis that IF-induced kidney dysfunction has the nature of tubulopathy is confirmed, in particular, by the results of the protein markers of renal functions analysed by us. Rats treated with IF were characterised with higher concentrations in urine and daily excretion of CysC, KIM-1 and NPH in the urine. Cystatin C is a new alternative for creatinine in terms of glomerular filtration markers [28]. This protein is an inhibitor of endogenic proteases responsible for the catabolism of multiple peptides, produced by all nuclear cells and released at a constant rate to blood. CysC, as a low-weight cellular protein, undergoes glomerular filtration and, subsequently, endocytosis in a mechanism dependent on the megalin receptor as well as irreversible degradation in the proximal tubules. Therefore, CysC concentration in the blood depends on the filtration rate of this protein, whereas in physiological terms, only low amounts of this protein are found in urine [29, 30]. The blood concentration of the classical glomerular filtration marker - creatinine - did not differ significantly in all investigated groups, which suggested a normal filtrating function. Thus, it can be assumed that increased CysC excretion in urine shown in our experiment could prove the impairment of tubular catabolism of this compound, constituting a premise supporting the hypothesis regarding the development of post-ifosfamide tubulopathy in the study rats. This hypothesis is also confirmed by the analysis of the results of the next evaluated compound – KIM-1. It is a protein physiologically present in urine in trace amounts (thus, its concentration value is expressed as [pg/mL]). KIM-1 is an ectodomain of a large transmembrane protein, which is a phosphatidylserine receptor of proximal tubules, mediating phagocytosis of apoptotic and necrotic bodies. Various toxic or ischemic factors, damaging renal tubules and interstitial tissue and leading to the development of acute kidney injury (AKI), cause an increased expression of the receptor, accompanied by a parallel increase in the concentration of KIM-1 in the urine. In the course of AKI, the surviving proximal tubules phagocytose debris of exfoliated cells, using the above-mentioned KIM-related receptor. The clearance protects the obstruction of the tubular lumen and infiltration by inflammatory cells, thus decreases the tubular hydrostatic pressure and reduces the risk of acute renal failure [31, 32]. Urine KIM-1 is, therefore, a good indicator of kidney damage and the protein itself, along with CysC, is one of the elements in the panel of laboratory markers, which should be currently determined to assess kidney function [33, 34]. In the described experiment, urine KIM-1 concentration and excretion of that protein in urine was 4 times higher after administration of IF, which indicates damage to renal tubules, not visible at the level of classic optical microscopy. Moreover, IF treatment also led to structural damage to the filtration barrier, which is proved by the increased presence of another protein – NPH – in the urine of IF-treated animals. Nephrin is a structural protein of gap junctions in the foot processes of podocytes [35, 36]. These visceral epithelial cells are highly-specialized units lining the outer surface of the glomerular capillaries and that neighbor cells of the Bowman’s capsule [37]. Podocytes exert essential functions, which all depend on their unique structure: controlling and maintaining the selectivity of glomerular membrane permeability, co-formation of glomerular capillary structures, participation in remodeling of the glomerular basement membrane and endocytosis of filtered proteins in the glomerulus [37, 38].
The nephrine-dependent glomerular disturbances are a pivotal element of the early stage of glomerular proteinuria pathogenesis. The immuno-inflammatory processes involving the glomerular basement membrane alter the structure and consequently NPH function (and co-occurring protein - podocin). It contributes to the dilatation and unsealing of the slit diaphragm, leading to its disappearance, impairing the filtration barrier and inducing proteinuria [39]. In the adult patient population, NPH was mainly evaluated as an early-stage biomarker of diabetic nephropathy, nephrosis (including lupus nephritis) and preeclampsia, and it was demonstrated that the urinary amount of nephrine correlated with microalbuminuria [38]. Nephrine-related disturbances are also a pathogenetic element of the inherited podocytopathies. It is now recognized that the common feature in minimal change disease (MCD), membranous glomerulopathy, crescentic glomerulonephritis, collapsing glomerulopathy, focal segmental glomerulosclerosis (FSGS) diagnosed in pediatric patients is through podocyte damage and dysfunction, manifesting by early increased urinary nephrine excretion [38]. Moreover, nephrine NPHS1 gene mutations are also the most common cause of rare and severe congenital Finnish-type nephrotic syndrome, characterized by the enlarged placenta, premature birth and massive proteinuria that begins in utero [40].
Thus, it can be concluded that the relationship between NPH abnormalities and the podocyte dysfunction is well documented and currently indisputable and the increased amount of this protein in the urine should be considered an early prognostic biomarker of developing proteinuria.
In our experiment, the revealed amount of NPH in urine allows, therefore, to identify the early phase of glomerular injury, which extends the description of post-ifosfamide tubulopathy revealed in our experiment with glomerulopathy developing in parallel. Therefore, we can state that the post-ifosfamide form of kidney dysfunction induced in our experiment combined the features of both tubulopathy and glomerulopathy.
Employment of additional NAC treatment in group 3 was connected with the alleviation of histopathological disturbances in the urinary bladders as well as reduction of functional disturbances in kidneys found in animals treated exclusively with IF, as mentioned above. The urea and BUN concentrations in blood were significantly lower than in group 2 and reached values similar to those recorded in the control group. Similarly, concentrations and daily excretion in urine of all evaluated protein markers reached significantly lower values in comparison to rats in group 2. Thus, it can be concluded that NAC, administered in parallel to the applied IF, had a uroprotective and nephroprotective effect. The pathogenesis of the said effects is complex, with key antioxidative effects featured by NAC, which, due to the presence of –SH groups, is a direct “sweeper” of oxygen and nitrogen free radicals. This compound also regenerates endogenic glutathione resources and, thus, decreases the cell prooxidative status. NAC also inhibits multiple intracellular pathways (c-Jun N-terminal kinase, p38 MAP kinase, SAPK/INK, c-Fos pathways, as well as nuclear factor κB) regulating the expression of genes coding the proinflammatory and antiapoptotic factors [41, 42]. Therefore, intervening in the above-mentioned acrolein/CAA initiated mechanisms, NAC reduces IF toxicity.
The concept of NAC as a nephroprotector has already been mentioned in the literature [39, 40]. It was not our intention to demonstrate for the first time that NAC exerts a nephroprotective effect, but to broaden the knowledge of the pathophysiological description of kidney dysfunction in the course of IF-induced cystitis and to contribute to the discussion of the NAC role in kidney protection. Our experiment assessing the positive effect of NAC was, to our best knowledge, the first based on the analysis of new protein markers reflecting the kidney functioning, and not only on the analysis of a panel of classical laboratory parameters used in the routine kidney evaluation. In our opinion, it is the novelty of our study. Based on the results of the evaluated novel markers of kidney function, we confirmed that IF caused renal tubulopathy (expressed by increased urinary KIM-1 and CysC excretion), which has previously been mentioned [5, 23, 43], but we also showed that administration of IF lead to damage of the structural elements of the glomerulus (manifested by increased NPH excretion with urine). NAC treatment alleviated the urinary excretion of the aforementioned proteins, which confirmed the nephroprotective effect of the compound. Our results are consistent with the work of Chen et al. [44], who proved in their experiment that i.p. administration of NAC in the dose of 1200 mg/kg b.w. alleviated post-IF renal dysfunction, reducing hypercreatininemia and excretion of beta-2 microglobuline in the urine. Moreover, they demonstrated that the application of NAC resulted in increased activity of glutathione S-transferase and an increase in glutathione concentration in kidney homogenates. Our conclusions regarding the nephroprotective effect of NAC are, therefore, consistent with the results quoted above. However, it must be emphasized again that our results were based on the evaluation of the novel biomarkers of kidney function, and Chen et al. [44] concluded their findings primarily from the assessment of a classic laboratory panel of low-molecular renal parameters.
The increasing number of reports from experimental studies on the nephroprotective effect of NAC is also reflected in clinical data. Hanly et al. [43] in their review paper briefly and synthetically characterized the rationale for using NAC in patients undergoing chemotherapy with IF. They also described cases of paediatric oncology patients suffering from renal dysfunction during IF treatment, which was mitigated with parallel NAC administration, and it was again convincing support for the nephroprotective use of NAC. They concluded that taking into consideration the fact that excessive oxidative stress is an essential element of the pathogenesis of renal damage during IF treatment, NAC as an antioxidant and glutathione regenerating compound appears to be potentially useful nephroprotector in a clinical setting. Therefore, it should be expected that consistent premises from both experimental studies (including our one) and clinical reports will result in the official approval of the use of NAC as a nephroprotective agent in the near future.
CONCLUSION
1. Intraperitoneal IF administration of the total dose of 250 mg/kg b.w. divided into 5 doses in rats caused the development of inflammatory changes in the urinary bladder and functional disturbances in the kidneys of tubulopathy and early glomerulopathy character, as was proven by the increased excretion of CysC, KIM-1 and NPH in the urine.
2. In parallel to IF treatment, p.o. administration of the total dose of 1000 mg/kg b.w. of NAC divided into 5 doses alleviated the inflammatory changes in urinary bladders and resulted in reduced renal dysfunction, manifested as a reduction in excretion of the above-mentioned proteins in urine.
3. Thus, NAC had both an uro- and nephroprotective effect. Due to its effect, NAC can be considered an alternative for mesna, currently applied as a routine uroprotective agent.
LIST OF ABBREVIATIONS
AKI | = Acute Kidney Injury; |
BUN | = Blood Urea Nitrogen; |
CAA | = Chloracetaldehyde; |
CP | = Cyclophosphamide; |
CysC | = Cystatin C; |
IF | = Ifosfamide; |
KIM-1 | = Kidney Injury Molecule-1; |
NAC | = N-acetylcysteine; |
NPH | = Nephrin |
AUTHORS CONTRIBUTION
L. Dobrek – designed study, performed research, interpreted results, performed statistical analysis, reviewed literature, wrote the manuscript, prepared figures and tables
K. Nalik-Iwaniak – collected data, contributed important reagents’, provided care for animals during the experiment
Z. Arent – performed ELISA tests
ETHICS APPROVAL AND CONSENT TO PARTICIPATE
The medical experiment was conducted at the Experimental and Innovative Medicine Centre of the University Centre of Veterinary Medicine of the Agriculture University in Krakow upon receipt of consent of the II Local Ethical Commission in Krakow, Poland (No. 154/2017).
HUMAN AND ANIMAL RIGHTS
The study was conducted in accordance with Directive 2010/63/EU on the protection of animals used for scientific purposes and with the national, Polish statutory law - Act of 15 January 2015 on protection of animals used for scientific or educational purposes (Journal of Laws, 26 February 2015, item 266) and after obtaining a written consent of the II Local Ethical Committee for animal experiments operating at the Institute of Pharmacology of the Polish Academy of Sciences in Cracow (resolution No. 154/2017 of 17 May 2017).
CONSENT FOR PUBLICATION
Not applicable.
AVAILABILITY OF DATA AND MATERIALS
The authors confirm that the data supporting the findings of this research are available within the article.
FUNDING
None.
CONFLICT OF INTEREST
The authors declare no conflict of interest, financial or otherwise.
ACKNOWLEDGEMENTS
The authors would like to thank the veterinary surgeon Tomasz Huć from the Animal Pathomorphology Laboratory of ALAB Veterinary Medicine in Warsaw for his assistance in histopathological tests.